Nuclear Structure
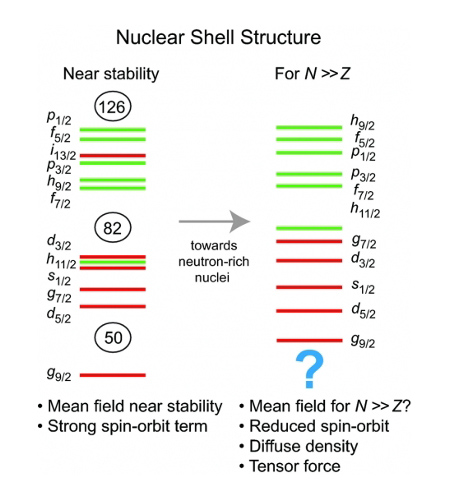
Figure 2: Nuclear shell structure with large gaps in the single particle
energy levels (left) is well established in nuclei near stability. This shell
structure is expected to evolve in more neutron-rich isotopes,with
important implications for both nuclear structure and the synthesis of
the heavy chemical elements in explosive astrophysical events.
Image source: www.nscl.msu.edu/media/images/isf
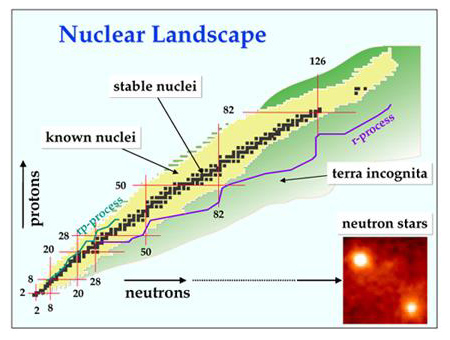
Figure 3: Schematic of the nuclear landscape in which each pixel
represents a particular number of protons and neutrons in the atomic
nucleus. Slightly lessthan 300 stable isotopes (black pixels) occur in
nature, while there are believedto be some 6000 bound isotopes
(green region), the majority of which have neverbeen produced or
studied in terrestrial laboratories. These radioactive isotopesplay
crucial roles in the synthesis of the heavy chemical elements in
explosive astrophysical events such as supernovae and neutron
star mergers.
Image source: www.orau.org/RIA
The atomic nucleus is a strongly-interacting, many-body quantum mechanical system that exhibits a fascinating variety of shapes and excitation modes, from spherical to super deformed (axis ratio 2:1), and from excitations of single protons and neutrons to collective vibrations and rotations of the nucleus as a whole. The study of nuclear structure attempts to elucidate the unifying mechanisms by which these rich patterns of behaviour emerge from the common underlying strong nuclear interaction between the nucleons (protons and neutrons) that form the nucleus.
Central to these studies is the concept of nuclear shell structure, in which protons and neutrons occupy quantized energy levels within a potential generated by their interactions with all of the other nucleons. As shown on the left side of Fig. 2, these quantized energy levels cluster into groups, or shells, and the filling of major energy shells leads to particularly stable configurations associated with the proton and neutron "magic numbers": 2, 8, 20, 28, 50, 82, and 126. Nuclei near closed shells tend to have spherical ground states, while those far from shell closures are deformed and exhibit low-energy collective rotational excitations.
While the nuclear shell structure and magic numbers have been well studied close to the "valley of stability" defined by the ~ 300 stable isotopes found in nature (black pixels in Fig. 3), investigations of exotic nuclei with unusual proton-to-neutron ratios are revealing an evolution of this shell structure (right side of Fig. 2) as one moves away from the stable isotopes. Understanding of this complex evolution of nuclear shell structure is of fundamental interest both as an emergent property of the nuclear quantum many-body system and as an important input to understanding the nuclear reaction processes by which the heavy chemical elements have been, and continue to be, synthesized in explosive astrophysical environments. As shown schematically in Fig. 3, nuclear shell structure in extremely neutron-rich nuclei plays a major role in the pathway for the rapid neutron capture (r) process, thought to be responsible for producing approximately half of the elements heavier than iron. Neither the exact locations of the shell gaps in these neutron-rich isotopes, nor the exact astrophysical events in which the r-process takes place, are, however, definitively established at present.
Gamma-ray spectroscopy provides powerful techniques to study nuclear shell structure through measurements of gamma-ray energies and angular correlations in low-energy decay experiments and through measurements of electromagnetic transition matrix elements between nuclear states in Coulomb excitation experiments with accelerated radioactive ion beams. Our group at the University of Guelph leads experimental studies of the evolution of nuclear shell structure and collectively throughout the nuclear landscape employing both of these complementary techniques. The GRIFFIN Spectrometer and its auxiliary detectors support our decay spectroscopy studies at ISAC-I, while the TIGRESS spectrometer is used in our experiments with accelerated radioactive beams at ISAC-II. The DESCANT neutron detector array is used for gamma-neutron coincidence studies in conjunction with both TIGRESS at ISAC-II and GRIFFIN at ISAC-I.
The most recent results from our group's nuclear structure research programs can be found in our publication and theses lists.